⚛ My chat (+transcript) with TAE Technologies CEO Michl Binderbauer on the future of fusion
Faster, Please! — The Podcast - En podcast af James Pethokoukis
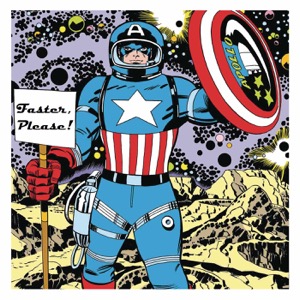
What if there were a way to generate massive amounts of affordable, carbon-free energy with minimal environmental or safety risk? Sounds too good to be true, but nuclear fusion just might be the kind of energy source that America—and the world—has been waiting for.Michl Binderbauer is the CEO of California-based TAE Technologies, a company trying to develop an aneutronic commercial fusion reactor. Michl joins us on this episode of Faster Please! — The Podcast to explain how his team is trying to make fusion power a real thing.In This Episode* Fusion’s Moment (1:11)* The Technical Challenge (12:11)* The Economic Challenge (15:33)* The Role of Government (22:20)Below is a lightly edited transcript of our conversation.Pethokoukis: What is sort of the current state of your company's technology, and in describing that, could you tell me how it sort of differs from other approaches in the field, keeping in mind I am not a nuclear physicist?Binderbauer: Understood. Alright, well it's a great introductory question. So TAE has been around, as you probably have read, for a good two decades plus, but the 25 year anniversary was just this past April, actually. We're at the stage now, it’s really exciting, where the machine we're under construction on now, which we call Copernicus, which is our generation six, is actually intended to get us to a point to demonstrate that we can harvest more energy than we have to feed it. And this is on a really engineering comparison, how much energy comes into the site and deploys on the machine versus how much can you harvest. To be fair, this is not a full power plant, so we're going to measure the heat output, the collective heat output on it. Now that's where we're going, and that's really enabled by 20 plus years of a journey of, interestingly enough, a lot of scientific nuance discoveries, but mostly technology development.What you learn is that the journey that we were on was mostly one of underestimating the complexity of power supplies, vacuum systems, heating systems in the form of us, this means energetic particle beams, and the technological tool chest around those things and making that work as a symphony, as a nice orchestra to do what we need it to do, and that's really where we spend most of the time, and now we're at the point where there's a confluence in understanding the science, understanding or having full practice capability, mastery of the tools, bringing these two things together in the sixth generation machine to drive net energy output. That's the goal. The other thing you asked me was how do we differ and to kind of contrast that a little bit?Because this is a very interesting moment for fusion, broadly, which are a number of startups, of course some of my listeners might be familiar with the breakthrough from the National Ignition Facility, which isn't really meant to create a nuclear power plant, but it was a great proof of concept that we can do some sort of fusion here. So I guess in a somewhat understandable way, given my own personal limitations, what are you doing that's sort of different than maybe some of the other companies such as, I mean I've written about Commonwealth Fusion and a few others, as well.Of course. Let me start by saying that, for most of that I should give credit to my brilliant PhD mentor who was a technical co-founder and co-founder in general of TAE. Norman Rostoker was his name, and Norman had an illustrious career in the field of fusion science and, in fact, accelerators and a few other areas of physics. He was a sort of polymath and really broad guy, which probably was a critical ingredient to get to where we are today. And so while he was very instrumental in the early days of the field in putting together a lot of the fundamental theory and things that I always joke and say, “You can't get a PhD in this field without suffering through a lot of the stuff he discovered.” But he also was very critical at the later stage in his career and he looked at this and said, “If we want to build something that caters to power production in a civilian way with good economics and the right kind of maintainability and practicality, then maybe what we're doing as a field today on the large sort of federal or national program-funded research was sort of missing the mark a little bit because it was building towards the Tokamaks, which some of your readers may know, those donut-shaped machines, the biggest of which is under construction in the south of France right now, it’s a big international project. And Norman looked at that and said, “That can get us to maybe net energy but not necessarily practical net energy or economic net energy.”In the end it's about an applied end product that we're going after, not textbook knowledge, in a sense, or a proof point for a laboratory experiment. With that in mind, when the company, before it even started—this is in the early ’90s when I became a student—he had a very delineated philosophy of end in mind: Let's look what this needs to look like. And that's pretty trivial to define, right? If I ask you, what do you think a good power plant should look like, you could probably tell me. If we can make it non-polluting, great, we want to make sure that it doesn't have maintenance every day. It's up most of the time and it can compete with what the grid needs today in terms of economics, who else makes power with from coal, the gas to whatever else. And that's kind of how we started, we said that would be the ideal reactor, and now how can we cater to that. And what is the gap if you reverse engineer from there to today that you have to fill? And that's really where we started and that led to a remarkably different trajectory.One of those, the first one, frankly, was fuel, right? When you think about tritium, which is the conventional goal set, and that's a fuel that's heavy hydrogen, when you “burn” that, quote-unquote, you get neutrons, which we know from fission, those are what propagates the fission process, and if you have a lot of neutrons, you get radioactivity. And tritium by itself is also used in our warheads. It's not the ideal material you loose in a civilian setting, it's typically classified, et cetera, so there's all these headaches and there's very little tritium, by the way, to go around. There's like 50 kilograms of free tritium in the world, and that's super expensive, something like $30,000 a gram or so is what's usually quoted. So there's a lot of handicap there if you want to turn that into an economic prosperous thing. And so we said, “Alright, well, what else is terrestrially possible?”And so not to be philosophical and say God gave us a very narrow bookshelf, but it kind of is. On one end you've got the neutronic fuel cycle with tritium, and then on the other end of this small bookshelf you have hydrogen and boron which are copiously available, both. There's no radioactivity to go in, and by the way, when they burn you get three helium particles, which is where our initial name came from, Tri Alpha Energy, we call helium particles in nuclear physics alpha particles. And so you look at it and you say, “Oh, that's pretty good!” I don't have radioactivity as a byproduct, I don't have to worry about shielding, I don't have high costs associated with those things. And by the way, if you look where boron is used today, it's dirt-cheap commodity products, it’s detergents and soaps and cleaning products and things like that. So, in a way, it fits the bill.Now its big handicap is it needs a higher burn temperature to cook.Very hot.Yeah. You look at tritium on one end, that's about a hundred million degrees, which already sounds insane, but keep in mind, as a physicist, we sort of define that as just the energy state in that material beyond the gas. We call these plasmas. This plasma is at a hundred million degrees for tritium. If you want to burn boron, you need about a billion degrees. Now that sounds absolutely crazy, but it's not the stove plate hot of a solid. It's a very few particles that get to zip around in the container at very high energy, and that gets you to that definition of eventually a billion degrees.By the way, for reference, the big Hadron Collider at CERN, the LHC, that actually makes charged particle clouds with temperatures up to five trillion degrees. So we can actually do this. Amazingly, humans have a technology base to actually do that. So we started with the idea of if we wanted that fuel cycle, we've got to find the container and the process that can hold that together and create those energetic states we need. And that led us ultimately to what is referred to as a “field reversed configuration,” and I won't bore everybody with the detail of that, it's a mouthful to begin with, but it's a very interesting magnetic container.I will say that much, that instead of, in the case of most other confinement systems where you have a lot of magnets on the outset—and by the way the magnets are a big cost component in a reactor, they're superconducting, they're large in scale, complex to manufacture—and in this case, in the FRC, most of the field is actually created by the plasma itself.So plasma is discharge particles, if they flow, they create a current and the current can make a magnetic field, and so the plasma can self-envelope with a magnetic field that it generates from its currents and that can help, believe it or not, hold it in place. It sounds kind of perverse, but it works. And the idea behind that was derived about 50 years ago—almost everything infusion had some origins back many, many decades ago—but it was always considered too finicky to make work because one thing you can appreciate, if there's anything wrong in the flow in the plasma, well then the fields start to deteriorate so it can very quickly get into negative feedback cycle unless you can keep it stable and well controlled.And that's what we developed now. So now we have this perfect incarnation of it where we can run at will for as long as we want. We control this with active feedback today with extremely fast circuits and very smart software that's machine learning based, that self-corrects, recognizes patterns, and stuff. So take it as a supposition. Now we have ability to make these field reversed configurations at scale, meters in size, and can hold them steady as long as we want. And now what's interesting about that container is that has a much easier scalability, from a physics perspective, to those high energy conditions. This is why it's the right container to marry with the hydrogen boron, and most people just didn't go there for two reasons, I would think.One is that when the field works mostly on, say, Tokamaks, then there is so much knowledge base developed there that, in a way, it self-propagates and the incoming young people that get graduate education, they work on what's the most prolific thing, which happen to be Tokamaks. So it sort of self-propagates.And the other thing, of course, is people felt that confinement or the ability to hold this material together is already really stressful, and so if you have to go to a hundred million degrees, let's try to celebrate there before we bother going further. Norman was more maverick and said, “But that's not a good endpoint to be, so why don't we shoot for something a bit more out there that can really bring all that together. As a graduate student, I was game for that. I thought this was a brilliant idea. It appealed to me enormously to say let's connect what we're trying to do to the applied end product, and that's where we started, first in the university and then built the business.neutral beams, the plasma maintains lasting, high-temperature field reverse configurations.The Technical Challenge (12:11)Where you are right now, what is required to get to the endpoint, which is a commercial reactor? Does that require continuous incremental progress and success, or does it still require something that you might call “leaps” in the technology? Where are we to get to that endpoint?Of course I should also explain that temperature increments like you're walking up a ladder, there's different steps to it. At the step where we are now, we're operating today the machine, the generation five—which we actually call “Norman” by the way, in its honor, we named it Norman. The reason that was fitting is because it established the scientific proof that you can actually create stable, long-lift field reversed configurations with the right attributes, and today we're doing that about 75 million degrees in the current machine that runs every day about 50, 60 experiments. So we know we can scale, we're really sure we can scale this to a hundred million degrees. What gives a hundred million back to the fuels, that doesn't give you boron but that gives you tritium. If you think about an approach of sequences, is you ramp up to a billion degrees, somewhere you have to cross a hundred, so you have something harvestable there from an economic opportunity.And so Copernicus, the next machine generation six that I alluded to earlier is the machine that's going to enable us to get into the tritium-level regime. This machine is going to show net energy capability at the a hundred to 150 million degree mark, which is typically where people operate with tritium. We were slated to do that by about ’26, somewhere late-’25 into ’26, and that's what we're constructing and fully projected to do. Now assuming success on that—which I believe is very much in our favor, we've been less than a factor of two of those operating conditions already and we have the engineering and the mastery, the operational mastery on this in hand—then the next step after that is to scale that up and build a machine that's about a factor of eight or so up in energy, and that gets you into the regime of the boron operation, and that's the stage when we think we will have net energy demonstrated out of hydrogen boron, and that's probably early 2030s.So again, coming back the next three years, make a machine that gets into the tritium equivalent operational regime. One thing I should point out, perhaps people may question, are we using tritium in the machine directly? We are not. What we're doing, and I can get into why that is, Commonwealth for instance—you mentioned earlier Commonwealth Fusion Systems (CFS)—they're trying to be a bit more ambitious and build this Tokamak and eventually fuel it with tritium. That has a much larger price tag and operational complexity because tritium is not an end game for us. I just want to enable the energetic state to burn tritium without actually doing it. The field has today sufficient enough confidence and maturity to understand that if you can hold the material together at a hundred, 150 million degrees with the right density and everything, yes, you could make tritium-based net energy if you wanted to, and that'd be for somebody else to do, but TAE wants to march on and build its boron reactor.The Economic Challenge (15:33)So there's a technical challenge that you feel like you're on track and you're sort of hitting some milestones there, and then there's sort of the economic challenge that we just don't want to get this thing to work, you want to get this thing to work so it makes sense that someday this thing can get plugged into an electrical grid. How do you feel about that aspect? How pricey or inexpensive or expensive will this energy be, assuming the not-insignificant technical challenges are met sometime in the next decade or what have you?Yeah, so great question. Obviously, we haven't built one yet. We haven't built the prototypes yet, let alone the full power plant, but there is actually a quite sane projection forward to those cost points from the fact that, after 25 years of working on this, we have a pretty good sense what we need. We have a great supply chain and partnerships established with people who built, not exactly this, but things like this. So you can do some estimates and you also know, as I said earlier, magnets are one of the biggest items, so there's a large amount of cost in there. The other big item that between those two controls more than two thirds is the heating equipment, and that can be radiofrequency heating like a microwave basically, or what we mostly use is injecting highly directional beams of atomic particles, neutral atoms that come in and then they collide with the fuel and then they basically transfer the energy that we directly shoot in and it becomes heat in the machine.So those two things, the heaters and the container system, the magnets, are the big expense items. When you get a sense for where you need to be and what the geometry looks like and so on, you can actually make a reasonable estimate at cost, and so I'm saying this, at the same time I'm asking for forgiveness if we're going to be off because obviously, in the end, there'll be a lot of detail that'll add to that. But I think what we believe, and I have confidence that that's correct, that the first generation of plants coming out of this, let's say it's somewhere in the mid-2030s, we'd have the first commercial plants installing and not plant one, which is a hand-built one-of-a-kind, right? But if you built maybe tens of plants, you will be at a point where you have some learning curves that bring prices down, you kind of know now how to do it. I wouldn't say it's mass produced yet, but it's going into a more efficient production cycle. I think we will slot in somewhere in the midfield today of generation assets.So if you're looking at solar, wind—solar maybe more than wind today—but you also add things like gas in the US, those are on the low end of the economics in terms of what they call LCOE, the Levelized Cost of Electricity, and then if you look at the upper end, you will find nuclear and the things where there's a lot of safety margin built in. We’ll be somewhere in the field in between there. That gets you pretty competitive right there because it has two other incredible attributes. One is that there is really no variability in fuel costs because it’s literally free because you need so little. Fusion is super high energy dense, so you don't need much.And the other aspect is that it doesn't pollute, really. There's no carbon involved, there's really no radioactivity to speak of. And so you are ending with something that can be baseload power, that's dispatchable, as they call it today. The human controls when it's on and when it's not, not the sun or the wind. And you have essentially green energy. In that sense, even if it's more expensive than some of the cheapest things today, but it's midfield, it'll be very competitive on a global basis and it'll be an important component that the world will need.How big a facility would you need to power Cincinnati or Chicago? My only experience is looking at the rather big nuclear fission reactors which are fairly big, so would this be a lot smaller than that?Well, two things: There's the machine size and then there's the installation size, the site. In nuclear fission today you have exclusion zone around the plant. There's a lot of the plot of land that it's on and then some incremental infrastructure, safety, security shielding and so on adds a lot of additional cost and scale. If on the boron machine, the actual machine is a couple, maybe three double-decker buses back-to-back, something like that, maybe a bit taller, but not that much. So that would be comparable to a large gas turbine, for instance. Or if you are in a hydro plant and you're looking at the generating units, they'd be sort of on that scale. So it's not outsized relative to what conventionally is used in the utility space today.Now if you look in the land footprint, it's pretty minimal. You're looking at a handful of acres at most. In fact, maybe even less over time. Now that's with hydrogen and boron because you then don't have radioactivity to speak of. You don't have the chance during an accident. The worst-case accidents that a plant like that would suffer would be industrial scale things. Things like a bad fire in a factory would just be similar, but it doesn't have nuclear meltdown capability. There is no chain reaction kind of thing, like we know from Chernobyl—and by the way, this isn't just true for TAE, this is true for all of fusion, it makes it really safe. So those attributes will shrink the site down. So if you're asking me how much can we get out of one of these systems at that scale, probably somewhere in the order of half a gigawatt, 400 to 500 megawatts is sort of what we're shooting for.It's a larger gas turbine system and if you wanted to get gigawatt-level power like you would get out of a fission nuclear plant today, you would probably have, say, two, three of these units next to each other. What I really think the world will go to and what we hear from talking to a lot of the utility people, it's a more distributed grid, ideally. You have things on the 300–500 megawatt scale that deploys in a way where you have more redundancy if you needed it, there's more reliability, et cetera. This is the scale that I think you would look at, so feeding a city like Chicago out of one plant, or the whole Chicago metropolitan area, is not going to happen. You would have a distributed set of systems, and you think 400 megawatts or so, you get a few hundred thousand households that run on that, and then you scale from there.The Role of Government (22:20)You've been working on this for some time and obviously I work at a think tank, so I always think, do you want government to do something that it's not doing? Do you want government to stop doing something that it's currently doing? I know I've certainly talked to some startups, newer startups, they're in partnerships with the Department of Energy. So what is your engagement currently and what would you need, or not need, from government going forward to get to where you need to go?That's a great question and one that has evolved. In the past, we've been purely privately funded and we built everything we've done so far on private capital and we're kind of the oldest of that. Now, as you said, there's a lot of younger companies in the space too, and I think this is great. We get more shots on goal and it makes us more valid. We’re not the only lone idiots out there. There's actually reason to believe that there's many smart people trying now, so that's a good thing.Now where we're going, though, is a stage where I think public-private partnerships actually start to make sense. When you look at the history of any kind of energy technology that came about, nuclear is sort of—I hate to use it because it seems like we're so similar, we're obviously very different in some ways, we share the taxonomy “nuclear,” but that's about it—but fission, if you look at the evolution, it gets subsidies. There's a risk offtake for the early plants that the government tends to shoulder. That can be a loan guarantee, it can be other kinds of financial arrangements, and then eventually it becomes commercial enough in the sense that people believe in the viability, they have a good sense for its reliability and so on, and then it just propagates into the market in a very capitalist free market sense. That transition out of the lab into that stage of really rolling out at scale I think is where we absolutely need to count on government support.In fact, what's wonderful to see now, over the last couple of years in particular, and you read about this, the White House last year had a summit where we and a few others in the front running in the private sector were there together with the national labs and people from DOE, and we had a very productive conversation about what the White House framed a bold decadal vision for fusion. Important in that is the recognition that it's not always 30 years away and always will be, it's much closer. And what can we do proactively and collectively to accelerate that?I think that's what's really heartening to see, that as we're getting to that proof point, now we're getting net energy and then we want to leap to a prototype and a power plant. We're going to need that help, and there is a public-private partnership around multiple things on the technology or production side, but then also moving into the ultimate: How do you fund and risk underwrite these early plants when utilities typically are more risk averse? That we see on the federal level, and then on the state level, I don't know if you follow that, but recently California and North Carolina had a couple of bills coming out—for instance, California, we've had this nuclear moratorium where everything nuclear in nature is sort of not tolerable, and that's been modified. Fusion now is excluded out of that and in fact is now part of what they would call the benign side of the future of energy. In fact, the California bill made it very clear that it's to be treated as clean energy, essentially. And so in North Carolina, so you have now a blue state and right state looking at and saying, we want this. We need this, and we recognize the chance is high that over the next decade this comes about.The other thing I can add is that the Nuclear Regulatory Commission, they just this year in a sort of landmark first step, the commission ruled in terms of where do we slop the regulatory framework around fusion. It's not in the old Part 50, which deals with the fission world, but it's going to be in a world that's much closer to where you would regulate medical accelerator that makes pet isotopes for oncology scanning and stuff. So they recognize that this is, while nuclear in taxonomy, it's a very different form of risk to the public, and therefore the level of regulation is lower, and that's equally important.The interaction with the government is super important now, and we're very heartened by the fact that we see there's really a nearing and a mutual excitement about bringing that out as quickly as possible against the backdrops from climate change to whatever else people are worried about with the national security and energy independence.Obviously, from my perspective, what I like is the idea that if fusion can succeed, it becomes eventually a source of abundance because there's so much fuel here that we can harvest and we think we can do this at very economic levels, that you can lift up those parts of the world that today are living on the other side of the gradient in a very sort of depressed, low quality of life. In fact, if you look at all the energy use projections or demand projections forward, you can see that we're going to more than double, and most of the demand comes from the underdeveloped world. Fusion can be a very big contributor to a more equitable world as a whole. It's all these attributes that I think get people really excited, and now it's no longer just this visionary dream. We're really, really close to doing it. I think that's why you see the government and everybody coming together now and beginning these earnest conversations over the next few years: How do we structure programs from regulation to working together to ultimately loan guarantees and other things in a public-private partnership, and bring it to the grid. This is a public episode. If you’d like to discuss this with other subscribers or get access to bonus episodes, visit fasterplease.substack.com/subscribe